Experimental Setup
The Deep Underground Neutrino Experiment (DUNE) is a leading-edge, international experiment for neutrino science. Discoveries over the past half-century have put neutrinos, the most abundant matter particles in the universe, in the spotlight for further research into several fundamental questions about the nature of matter and the evolution of the universe — questions that DUNE will seek to answer.
DUNE will consist of two neutrino detector systems placed in the world’s most intense neutrino beam. One detector will record particle interactions near the source of the beam, at the Fermi National Accelerator Laboratory in Batavia, Illinois. A second, much larger, detector system will be installed more than a kilometre underground at the Sanford Underground Research Laboratory in Lead, South Dakota — 1,300 kilometres downstream of the source. These detectors will enable scientists to search for new subatomic phenomena and potentially transform our understanding of neutrinos and their role in the universe. DUNE will utilise the latest in liquid argon time projection chamber technology.
A number of prototype detectors are located at CERN. The first started taking data in September 2018 and the second is currently in the commissioning phase.
The Long-Baseline Neutrino Facility (LBNF) will provide the neutrino beamline and the infrastructure that will support the DUNE detectors. Groundbreaking for the LBNF excavation and construction at Sanford Lab occurred on July 21, 2017.
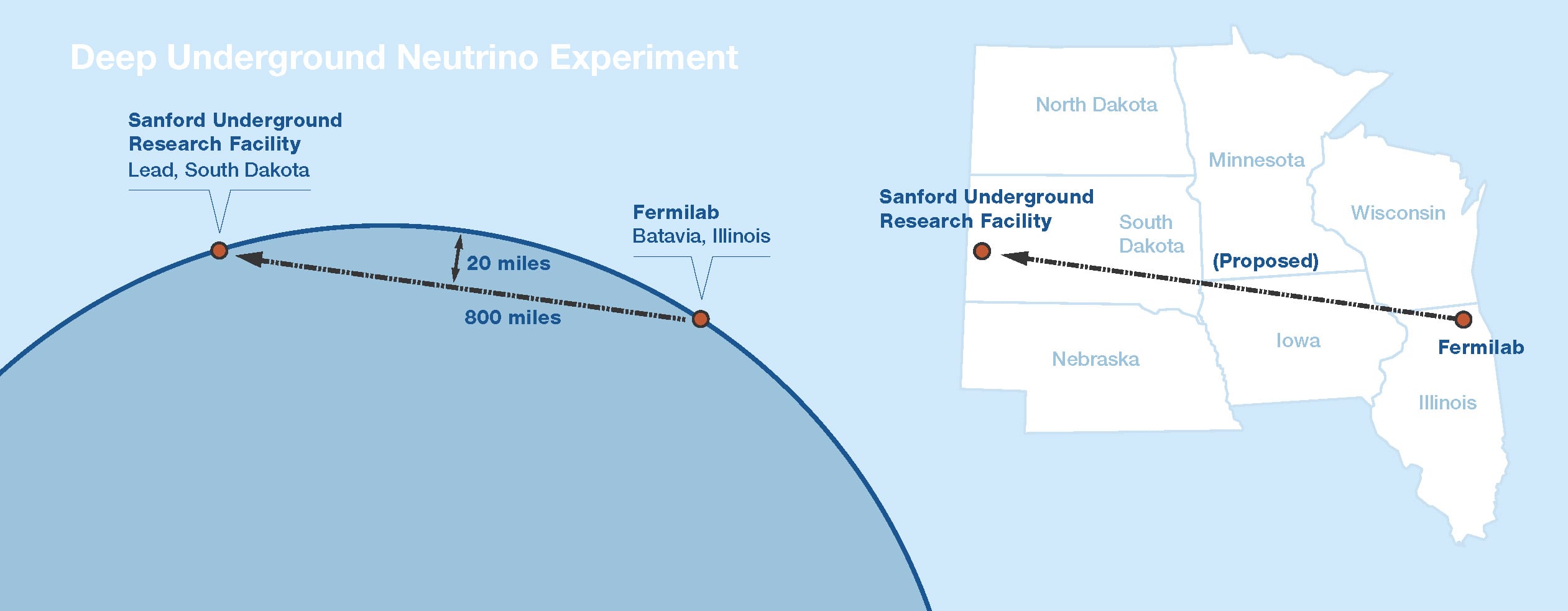
The DUNE Far Detector
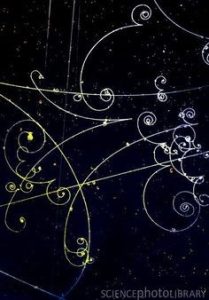
The DUNE far detector comprises 4 10 kton (~7 M litre) modules including at least two Liquid-Argon Time Projection Chambers (LArTPCs). LArTPCs provide 3D imaging of neutrino and other particle interactions in the vast volume of the detector with bubble-chamber-like resolution (~mm). Liquid argon is a cheap, dense, noble gas chosen to maximise the likelihood that we will record a neutrino interaction in a given detector volume.
When a neutrino enters an LArTPC and interacts, energetic charged and neutral particles are produced. As the charged particles traverse the detector they simultaneously ionise and scintillate the argon, producing electrons and photons respectively. The electrons drift to readout planes on one side of the TPC under an electric field which spans the entire detector. These readout planes are made up of either multiple layers of wires or pixels, which record the position and current induced by the ionisation electrons. Scintillation light is recorded by a photon detection system to provide additional timing and energy information about the charged particles. Together, the position, timing and energy data are used to reconstruct key features of the initial neutrino-argon interaction.
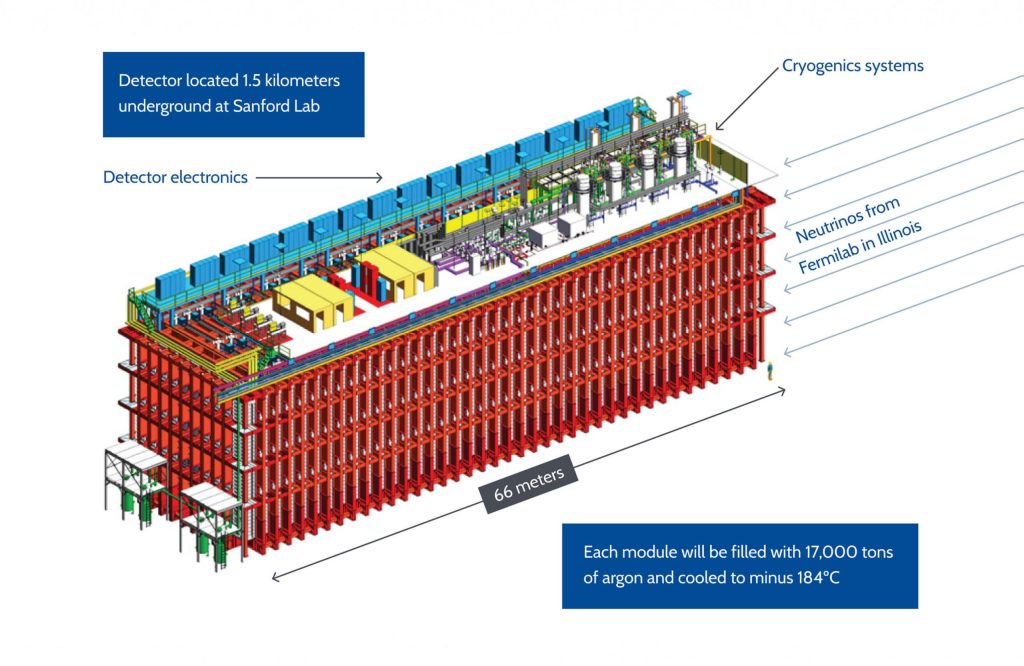
The DUNE Near Detector
The DUNE near detector is due to be formed of three main subdetector components, known as the ND-LAr, TMS/ND-GAr, and SAND. The beam will head through these in order, so each one can take specialised measurements before the beam moves on to the far detector. The ND-LAr is a LArTPC which will be the first of these detectors to see the beam. It will be able to take measurements both directly in the beam as well as at a number of angles to ensure detailed measurements of the whole flux range are taken. TMS/ND-GAr refers to the central detector. TMS stands for “The Muon Spectrometer”, which will be the day-one detector in place to get some initial measurements. This is not a TPC but is instead another type of detector made up of a large number of steel sheets. Once the technology has been appropriately tested, it will be replaced by the ND-GAr. This is a TPC which uses gaseous argon instead of the usual liquid, which provides a better signal-to-noise ratio. This detector can also move off-axis with ND-LAr. The final detector is the System for on-axis Neutrino Detection, or SAND. This will always remain in the main line of the beam to ensure everything is stable and provide constant measurements of the flux, but its exact format is yet to be finalised.
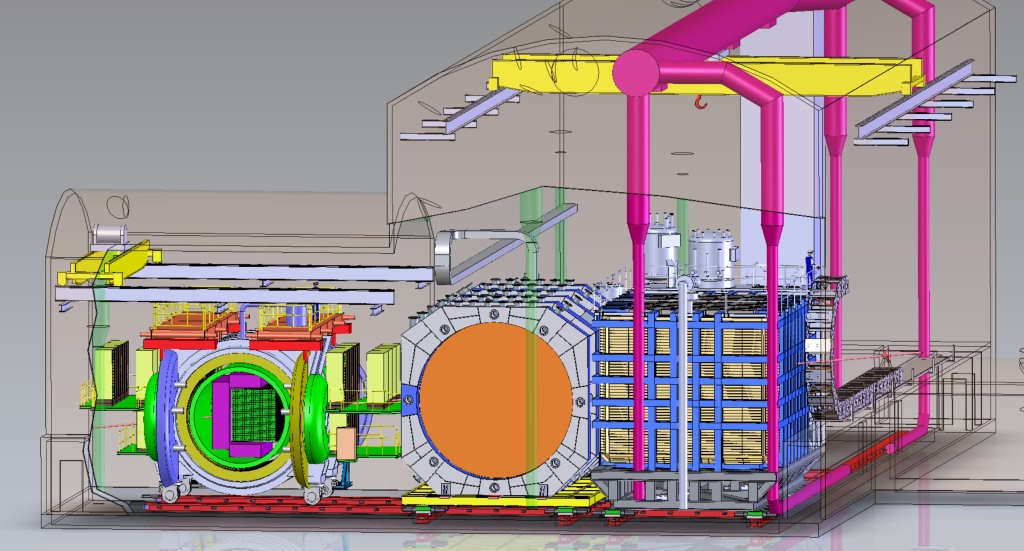
Neutrino Detection in LArTPCs
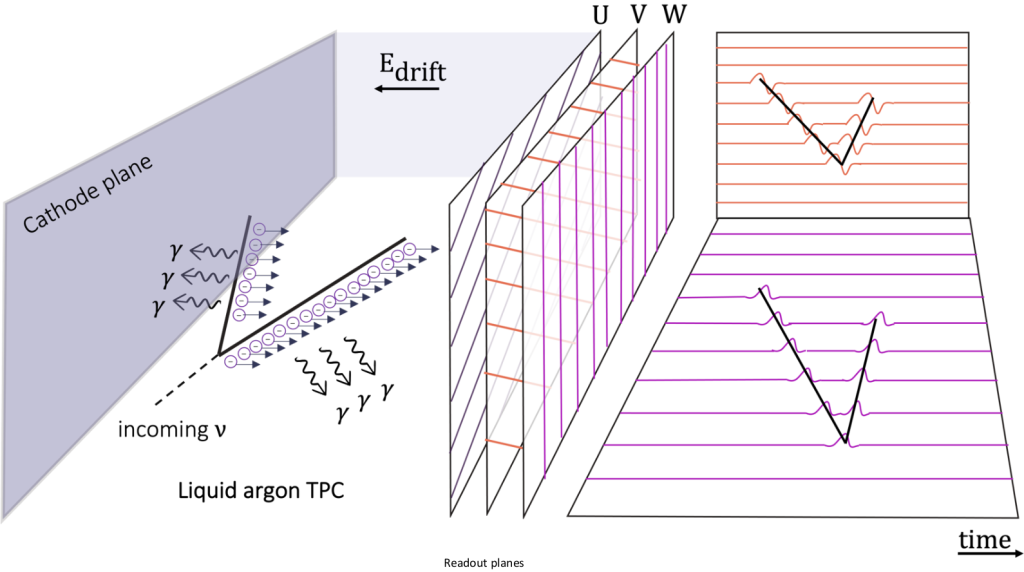
When a neutrino travels through our detectors, the fact that it has no electric charge means it cannot be seen. However, when it interacts with argon, it can produce new particles, including charged ones. As these travel through our detectors swiftly passing by argon atoms, they remove electrons from the outer atomic shells, leaving behind positively charged ions and a trail of negatively charged electrons as a tell-tale sign of their passage.
The goal of our detectors is to catch these electrons, which move at a constant velocity (about the same as the speed of sound in water), which is actually rather slow in relation to the particles that created them (which travel near the speed of light). Catching these electrons means we capture an imprint of the charged particles that emerged from the neutrino interaction. In order to do this, we create regions with a strong electric field inside the detector, so that the positively charged argon ions drift towards one end (cathode) and negatively charged electrons drift towards the opposite end (anode).
The anode consists of different planes of wires or strips, where the electrons create small voltage pulses when they reach them. These pulses are read out for each plane individually by an electronics system. The pulse time tells us how long the electron has been travelling towards the wire plane, and therefore how far away from the wire plane the electron was when its journey began, while the particular wire where the pulse was generated gives us a position coordinate. From this information we obtain a set of exquisitely detailed images, representing a different projection of the neutrino interaction for each plane, and when combining images from the three planes we are able to reconstruct the three-dimensional trajectories of the particles produced by the neutrino and learn more about the neutrino and its interaction with argon.
Other Neutrino Detectors
Yes! There are several neutrino detectors around the world, take a look at the image below to find out more about them.
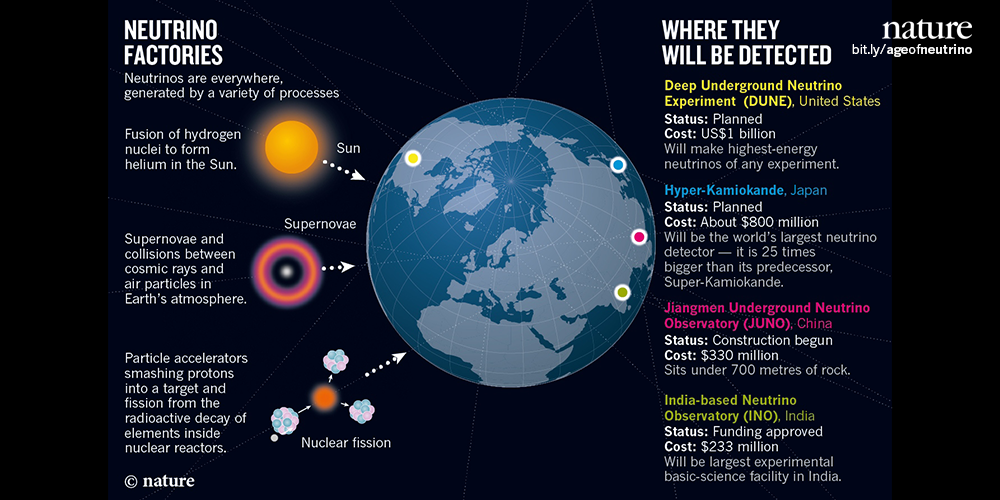